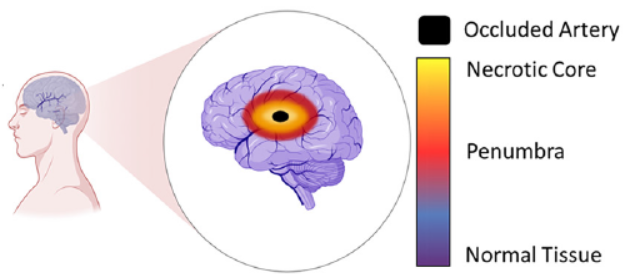
New research published in Lab on a Chip (September 21st) by researchers from the Ayuso lab (University of Wisconsin) and the Euan MacDonald Centre (University of Edinburgh) is an elegant example of a microfluidic organ-on-a-chip device. In this case, the organ is the brain, and the target disease is stroke. Stroke, or “brain attack”, is the brain equivalent of a heart attack. The majority of strokes are ischemic, typically caused by blood vessel blockage that leads to cell death in the brain tissue area served by that vessel.
The two research groups showed impressive results using rat astrocyte brain cells (which are a kind of “glia” — cells that support the brain’s neurons) to model the damage resulting from ischemic strokes, the response to reperfusion of nutrients to the deprived cells, and the viability of tissue grafting in an area damaged by stroke.
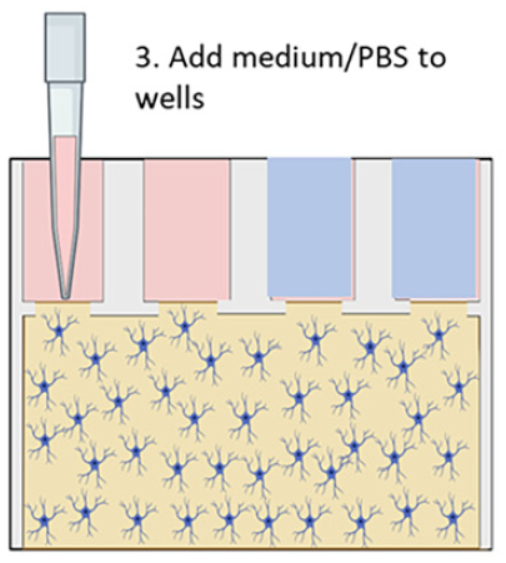
Their microfluidic brain-on-a-chip device was made from a 384-well microtiter plate with various bottom chamber configurations machined out via CNC milling, and sealed with a polystyrene laminate using double-sided tape or solvent-assisted bonding. The chambers were filled with a collagen gel/astrocyte suspension (a proxy to mimic brain tissue) through the upper well openings, and then covered by filling the wells with either a nourishing medium, to simulate normal conditions, or a simple phosphate/borate pH buffer solution (PBS) lacking nutrients, to simulate deprivation during a stroke; see Figure 2 above.
Experiments were performed to show that their simulated normal and stroke conditions over different periods of time were reflected in terms of cell viability. In addition, the extent to which cells deprived of nutrients and oxygen during a simulated stroke were able to recover when replenished with nourishing medium was explored. The schematic shown below at left in Figure 3 illustrates a simple stroke simulation experiment (E), while the microscope image at right (F) and below (G) show the resultant effects on the astrocyte cells. Live cells are stained with with a green fluorescent dye (Cell Tracker Green), and dead cells with a red dye (propidium iodide). Magnified views of the cells directly beneath, nearby, or far from the nourishing medium are also shown (G); the further from the medium, the higher the proportion of dead cells.
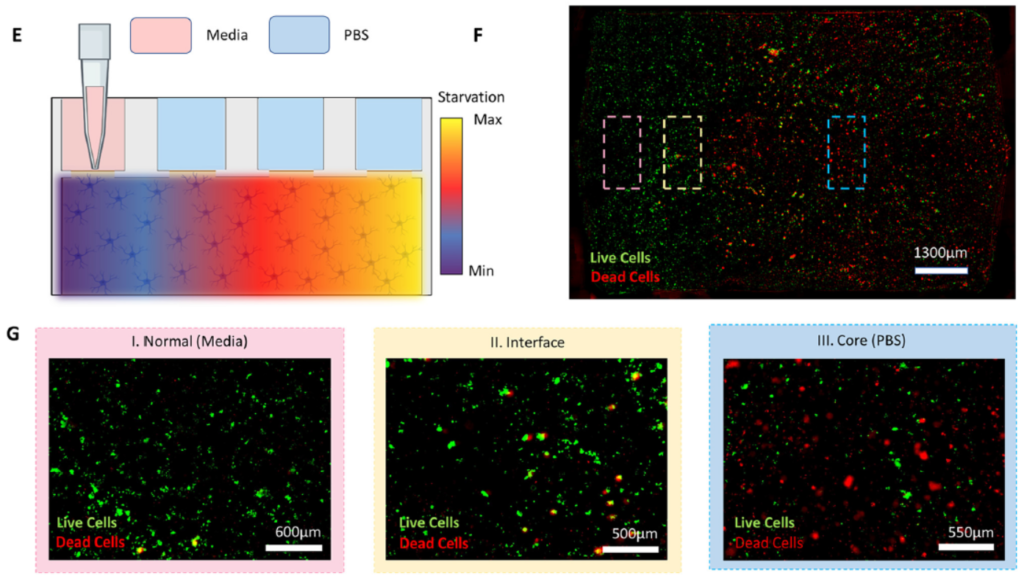
Adding different labelling and assaying approaches to the microscopy techniques above, they were able to model several other key stroke features. They demonstrated that reperfusion of the damaged pseudo-tissue area with nutrients from the medium after the simulated stroke did not reverse the damage done, replicating what has been observed clinically. Stroke damage was also evident in the deprived and reperfused cells vs. healthy cells by the dysregulation of certain genes critical to governing glucose metabolism; these experiments were performed by quantifying up- and down-regulation of genes using RT-qPCR on RNA obtained by bead extraction from the astrocyte-gel matrix at different stages of the experiment.
Lastly, and perhaps most excitingly, the researchers were able to show the brain-on-a-chip’s ability to potentially evaluate therapeutic treatment candidates. They punched a hole in the astrocyte gel matrix, added a suspension of macrophages (aka white blood cells; they are said to be “key players in the inflammatory response that follows stroke damage”) to the space, and imaged their movement over time with fluorescence and confocal microcroscopy. A schematic of the experiment is shown in Figure 4 below. Significant penetration into the surrounding pseudo-tissue was seen at 24h vs. 30 min.
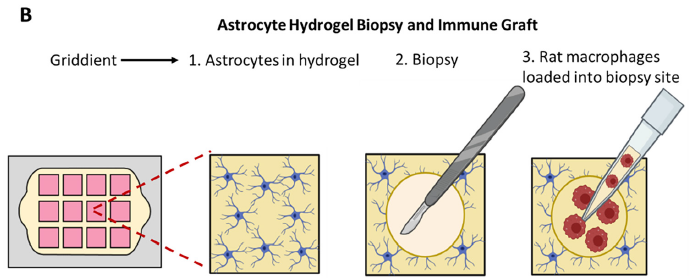
The importance of these results is significant. The research team has shown that it can monitor the health and location of astrocyte cells with a number of analytical techniques including live- and dead-cell fluorescent tagging, and monitoring of gene regulation. It has also demonstrated that their attempt at tissue grafting showed successful cell penetration. This at least means that their brain-on-a-chip device should be tested more rigorously to explore its capabilities on several fronts — for example, monitoring movement and viability of different cells within a variety of tissues/proxies, evaluating the effectiveness of emerging, unproven stroke therapeutic treatments, etc. Furthering this research could provide invaluable insight into stroke tissue damage and regeneration techniques. Also, there is no reason to think that a similar approach could not be taken with other tissue models to characterise diseases and conditions, and evaluate therapies.