New research from David Juncker’s lab at McGill University’s Biomedical Engineering Department show’s impressive progress along the path to sophisticated diagnostics or analyses performed on low-cost microfluidic devices in resource-limited environments. Their work was just published last month in Nature and also profiled in an article in McGill’s Health e-News.
Juncker’s group excels at capillarity-driven microfluidic devices, and they wrote an excellent critical review paper in Lab on a Chip a few years ago on ‘capillaric circuits’. It provides a full description of the physical chemistry of Laplace pressure and surface chemistry that underpin the capillarity-driven microfluidic operations used in these devices. While the full depth of the chemistry and physics is beyond the scope of this blog article (and the author’s RAM capacity ;-), they may be explored separately in a future post. Suffice it to say that, while somewhat involved, the capillarity-based pumping, valving and timing technology is well understood and and fairly well developed by this group, amongst others.
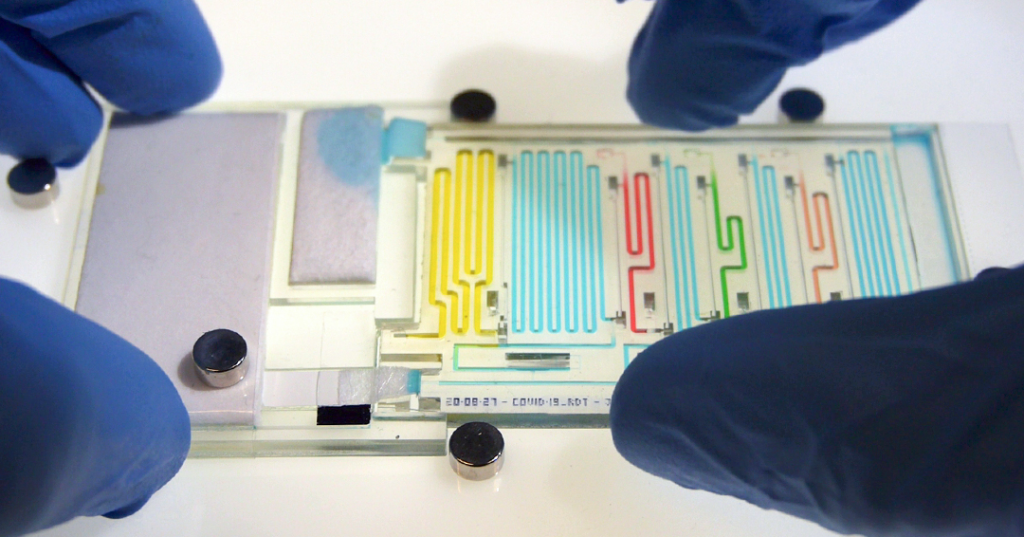
In this work, they are moving from the ‘mere’ sequential delivery of a few liquids at a channel intersection to fully configured, multi-step analyses using hundreds of sequential pre-programmed liquid manipulations. A first chip enables sequential delivery of 300 sample aliquots, while the second, shown above, performs the 8-step ELISA detection of COVID-19 antigen found in a patient’s saliva sample . Some impressive videos showing the performance of the 300 aliquot chip and the COVID-19 diagnostic chip can be found in the McGill article.
For someone like myself working in the field of microfluidics, perhaps the most thought-provoking aspect of this article is the potential for the technology to dramatically simplify the operation of fairly complex microfluidic devices. Being able to programme sequential volumetric flows with precise timing with a virtually unrestricted number of on-chip steps, all under autonomous operation, has far-reaching implications. It means that, after addition of sample(s), the device will simply start its protocol, with no interaction needed from an instrument or human operator. Using existing, fully developed on-board technologies such as lyophilised reagents and mixing procedures together with simple visible bands for detection such as with COVID-19 rapid tests, the door to many other more complex medical diagnostics, industrial or environmental assays is now open.
Development of products to meet these application needs will no doubt carry challenges, but at the end is a cheaply manufactured, complex device that can be used at the point of care or need; this represents a huge foundation on which to build any number of enabling, disruptive products.