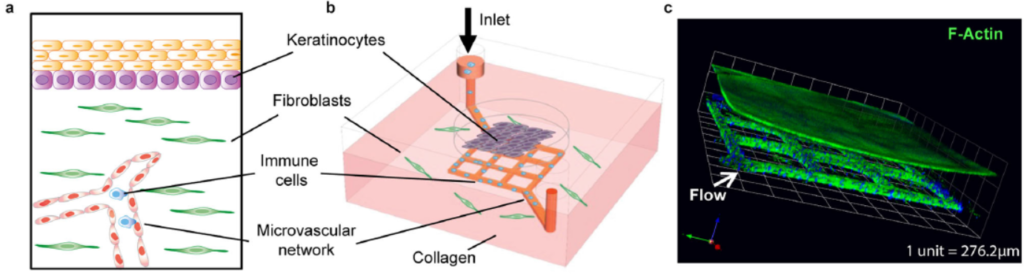
Impressive work out of the Zhu lab at the University of Washington’s Department of Laboratory Medicine & Pathology and Institute for Stem Cell & Regenerative Medicine appears to effectively mimic human skin by combining a microfluidic “blood vessel-like” vascular network and a stratified epidermis structure. The research was published in September in Nature Communications, and profiled by the UW’s Fred Hutch Cancer Centre.
The skin-on-chip device was constructed in several steps. First, a microfluidic network with 100 µm-wide channels was patterned via soft lithography/injection moulding in a collagen matrix with dermal fibroblasts. The channels were perfused with vascular endothelial cells that lined the surface and formed a vascular network. Second, at the outer surface of the collagen chip, the epidermis was constructed: epidermal keratinocytes were seeded for 3 days, and later exposed to air to differentiate into a multilayered epidermis. The graphic above shows diagrams and a confocal micrograph that illustrate the skin-on-chip device.
The growth of the seeded keratinocytes into a fully stratified epidermis over ~2 weeks was characterised with cross-sectional images and a variety of fluorescent dyes to show the growth of the layers. Comparisons of different fluorescently stained keratinocyte markers (K14 & K10), basement membrane markers (Col IV) and vimentin in the skin-on-chip model and in native skin showed the model to accurately mimic the natural structures in native skin.
Many experiments were performed to illustrate the abilities of their platform; two are showcased here. In one experiment, the effectiveness of herpes simplex virus 1 and 2 (HSV-1 and -2) infection was tracked at various stages of dermal growth, pinpointing dramatic decreases in infection rate with completed layers of dermal growth as days progressed. After just 1 day of exposure to air and differentiation of the dermal layer, the number of infected cells dropped from 67 to 16% for HSV-1, and from 100 to 36% for HSV-2. It’s no surprise that, as skin forms or heals, its ability to prevent infection improves, but accurately determining at what time, stage of growth and to what extent this happens via a real model is powerful indeed.
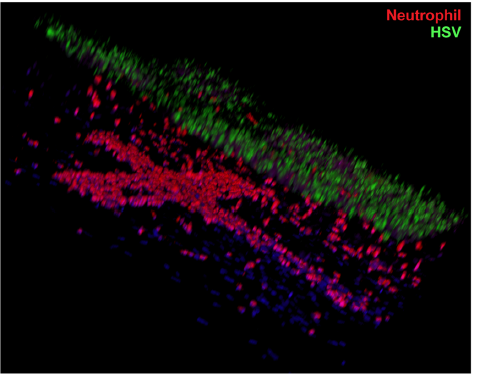
In another experiment, the authors showed that neutrophils (the most abundant type of the white blood cells used in our body’s immune response) would adhere to the walls within minutes of perfusion through the microfluidic vascular network for a chip that was infected with HSV, vs. essentially no adhesion in a control chip. After hours, the neutrophils continued to accumulate, transmigrated through the endothelium and entered into the layered dermis on the chip surface. A confocal microscopy image, reproduced at right, shows the presence of red-stained neutrophils in the cross-shaped microfluidic vasculature below, and migrating upwards towards the HSV-infected skin layers above, stained green.
The effectiveness and utility of this skin-on-chip model system to monitor immune responses to disease is obvious. The authors discuss an array of investigations that can be undertaken to better understand the chemistry of immune responses. If we consider the 3-D spatial information content provided by confocal microscope imagery in the context of their stratified, vascular-epidermal microfluidic system, it seems like the sky is the limit for recreating immune and other skin-based biological/biochemical processes, and perhaps with other tissue types as well. There is a good reason this paper was accepted by Nature Communications. 🙂